Achieving net zero is a critical global goal, requiring urgent action to reduce emissions and tackle the amount of carbon dioxide in our atmosphere. Removing carbon dioxide from the atmosphere is essential alongside cutting reliance on fossil fuels.
Innovative strategies to remove CO₂ include both nature-based solutions and advanced technologies, each contributing to a lower carbon content in our environment. The World Resources Institute emphasizes that reducing atmospheric carbon is key to combating greenhouse gases and creating zero-carbon pathways.
This article delves into a range of carbon removal methods, both natural and human-made, that essentially remove CO₂ from the atmosphere.
What Is Carbon Dioxide Removal?
Carbon dioxide removal encompasses a diverse range of methods to remove CO₂ from the atmosphere. The challenge lies in identifying methods that are not only effective but also scalable enough to have a meaningful global impact, without being overly resource-intensive or logistically complex.
For example, enhanced weathering crushes rocks to create more surface area exposed to oxygen, allowing them to release more minerals and absorb more carbon. However, on a mass scale, it is incredibly energy-intensive.
Carbon dioxide removal can either be natural processes, such as photosynthesis or ocean absorption, as well as to humanmade solutions, such as DAC (Direct Air capture), or BECCS (Bioenergy and Carbon Capture and Storage).
Why Carbon Removal is so Important
Carbon emissions are the primary contributor to global warming. They add layers of greenhouse gas to the atmosphere and trap heat, rising the planet’s surface temperature, causing extreme weather, rising sea levels, droughts, biodiversity loss and ocean acidification.
Scientists agree that reducing greenhouse gas emissions is essential to combat climate change, but significant amounts of carbon must also be removed from the atmosphere.
Natural Ways CO2 is Removed from the Atmosphere
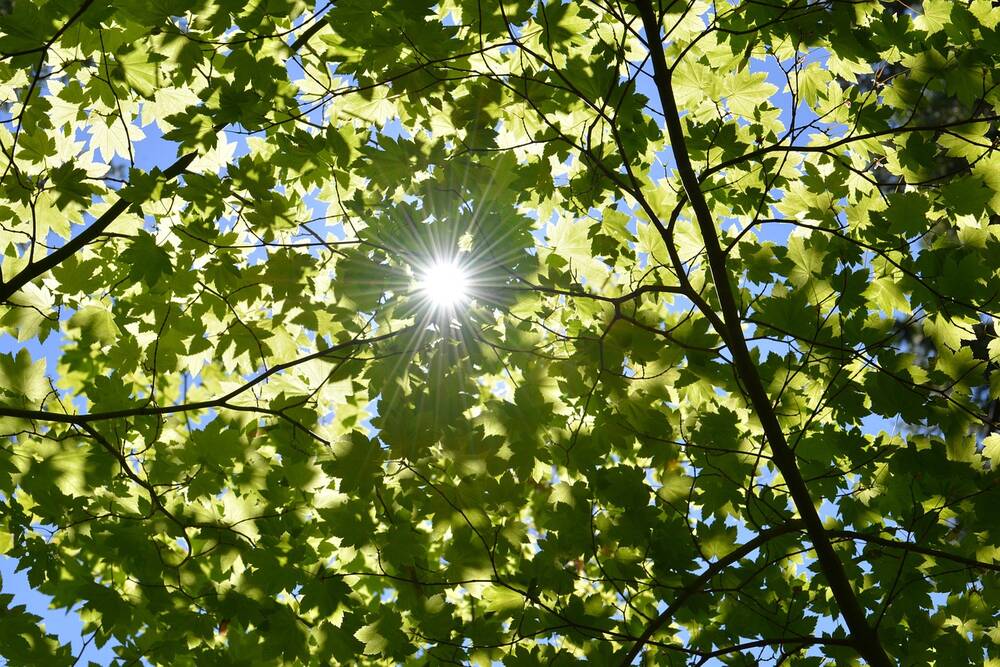
Photosynthesis
In photosynthesis, plants absorb CO₂ to convert it into glucose and oxygen. All plants store carbon within their biomass, with forests as carbon sinks, and the most significant forests in the world are some of our strongest mitigators against global warming.
We depend on photosynthesis for all life on Earth, but it alone is insufficient in combating climate change. It can’t keep up with our rapid rate of emissions, with severe rates of deforestation, pollution, and increased CO₂ levels disrupting plant growth and naturally occurring processes.
Ocean Absorption (via Phytoplankton)
Another natural form of carbon removal is ocean absorption via phytoplankton which occurs during photosynthesis. They are crucial for the long-term storage of carbon.
At the very bottom of the food chain, the phytoplankton are consumed by small marine organisms, and then larger and larger, spreading the carbon along a complex food web. When these animals die or produce waste, organic material falls deeper into the ocean. The deeper they go, the less decomposition there is, meaning the carbon is stored.
Beyond phytoplankton, a range of marine ecosystems can sequester vast amounts of carbon, such as kelp forests and seagrass meadows.
Decades of ocean acidification, warming ocean temperature, pollution, amongst other risks, have lowered the ocean’s capacity for carbon storage.
Soil Carbon Sequestration
An example of soil carbon sequestration are polycultures. They involve growing multiple varieties of crops together, mimicking a natural environment and creating biodiverse ecosystems with complex structures and services, resilient to external challenges.
Polycultures are much more attractive to pollinators, leading to increased crop yield, reduced pest populations, and enhanced soil health able to support healthier, stronger wildlife. More dense wildlife equates to more carbon stored.
Another example is crop rotation; when farmers regularly switch which crops are on specific fields. This routinely restores the nutrients in the soil, such as nitrogen. Microbial activity is enhanced, with different root systems releasing organic compounds that improve soil structure and fertility, leading to more carbon sequestration. Deep-rooted plants make soils resilient to erosion.
The microbial activity is enhanced, with different root systems releasing their own organic compounds which improve soil, sequestering more carbon.
Blue Carbon Ecosystems (e.g., Mangroves, Seagrasses, Salt Marshes)
Blue carbon ecosystems could be tidal marshes, sea grasses, mangroves, salt marshes—or any marine / coastal habitats that store carbon. Mangroves can store up to 10 times the amount of carbon than forests, due to their high productivity, anoxic soil conditions, long lifespan, and complex root systems.
These ecosystems must be protected for climate change, able to store carbon for thousands of years, while supporting a broad range of biodiversity and marine habitats.
Carbon Removal Technologies
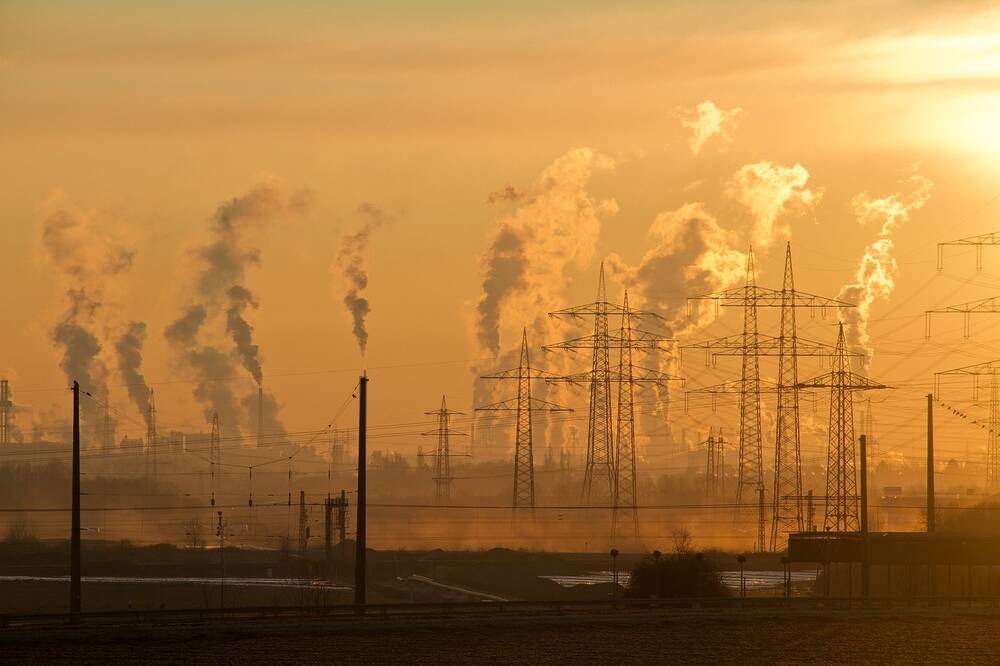
Afforestation and Reforestation
Reforestation directly combats deforestation by planting trees in areas that have been deforested, giving the forest a chance to take over again. This can be government-led or community driven, and allows for the long-term production of resources while enhancing biodiversity.
Afforestation, on the other hand, is the planting of trees in non-forested areas, creating new ecosystems that can convert degraded land, such as brownfield sites, into productive biodiversity hotspots.
Both techniques require regular monitoring and maintenance to ensure that the development is successful over time, however this lessens when trees become more established in the intermediate ages.
They are both essential strategies for carbon sequestration, as the more trees, the more CO₂ absorbed into biomass and soil.
Direct Air Capture (DAC)
DAC (Direct Air Capture) works by a process of air capture, such as fans, taking in ambient air through fans with chemical sorbets or filters, separating the CO₂ with chemical reactions, releasing the CO₂ with pressure or heat through regeneration, and then utilising the carbon dioxide in products or storing it underground.
Developing from academic discussions in the late 20th century, often credited to Klaus Lackner with the idea of capturing CO₂ from air in the late 1990s. The first development of practical DAC systems began in the early 2000s, with major advancements from David Keith. Moreover, Jan Wurzbacher and Christopher Gebald founded Climeworks, which brought DAC into commercial function in 2009.
DAC systems can be modular units the size of a shipping container, such as Climeworks’ or all the way up to large-scale plants, covering several acres from factories to industrial estates.
Through carbon accounting, many companies opt to offset their emissions with the purchase of DAC-based carbon credits. These are a verified carbon removal strategy, where each credit typically amounts to a ton of CO₂ removed (the equivalent of planting and growing 44 trees for a year / taking a car off the road for 10 months.)
The market for DAC credits is still emerging, but growing demand and supportive government policies are driving its potential. Standards like Verra or Gold Standard ensure credit validity, with primary sellers being Carbon Engineering and Climeworks on marketplaces like Puro.earth and Patch.
Bioenergy with Carbon Capture and Storage (BECCS)
Bioenergy with Carbon Capture and Storage (BECCS) combines bioenergy (BE) production with carbon capture and storage (CCS). Not only does this generate renewable energy, but it also actively removes CO₂, simultaneously addressing climate and energy goals.
This involves collecting biomass such as dedicated energy crops, agricultural residues, or wood, which absorb CO₂ during growth. It is then harvested and converted into bioenergy through burning (and sometimes gasification or fermentation), whereby it can be used to generate electricity or produce biofuels.
- BECCS projects can resemble power plants, with combustion chambers or boilers to burn the biomass, carbon capture units in the form of towers or columns to separate exhaust gases and CO₂, plus compression equipment or pipelines for storage.
- BECCS was first conceptualized in the 1990s, piloted in the 2010s, and today full-scale plants operate but in a limited capacity—such as Drax Power Station, UK, and ADM Decatur Facility, USA.
- The UK and USA government fund BECCS with climate policies, with organisations like the IPCC (Intergovernmental Panel on Climate Change) recognising it as a potential tool to limit global warming.
- BECCS credits exist, usually representing a ton of CO₂ removed, and must be permanent and verified to be certified.
Unfortunately, it requires vast land use, high capital and operational expenses, and energy intensity (with biomass cultivation, transportation, and processing). BECCS sometimes uses fermentation, producing pure CO₂ emissions from biofuel creation as a byproduct, causing direct carbon capture. Moreover, instead of burning, gasification turns biomass into syngas, used for cleaner energy production.
Ocean-Based Carbon Removal (e.g., Algae Farming)
The ocean not only holds 42 times more carbon than the atmosphere, and also covers 70% of the earth. Ocean-based carbon removal (OBCR) leverages this vast resource by capturing CO₂ through large-scale farming structures on top of the ocean. These hold seeded algae such as microalgae or kelp, covering up to several square kilometers of ocean surface.
Through photosynthesis the algae absorbs CO₂ during growth, which is then stored as biomass when they die or sink. At the lower ocean levels, decomposition is slower, meaning it takes a lot longer for the carbon to be re-released into the atmosphere.
Algae captures more CO₂ than terrestrial plants as it has higher photosynthetic efficiency and rapid growth rates, while not requiring arable land, making it sustainable.
Beyond storing carbon, the algae can also be harvested and utilised for biofuels, animal feed, bioplastics, pharmaceuticals, cosmetics, and fertilizers, amongst a range of uses. This provides economic value through revenue streams, making algae farming attractive to investors and financially sustainable.
Currently in experimental phases, the largest project is currently developed by Brilliant Planet, covering 30,000 square metres in Morocco’s coastal desert. The project has been going since 2013, and they are now working on a scalable production for global deployment, with the most optical locations in Southeast Asia, as well as the Indian and Pacific ocean.
Carbon credits linked to this method are relatively new, but can be purchased.
Enhanced Weathering
Another form of OBCR, enhanced weathering accelerates the natural process of rock weathering by crushing rocks to increase their surface area, exposing more minerals to react with CO₂. This reaction forms stable carbonate minerals, effectively removing CO₂ from the atmosphere and storing it in the rock. These carbonates can remain stable for thousands to millions of years, making it a long-term solution.
The broken down rocks can be spread through forests, on croplands, or on barren soils that can use the minerals. Soil health gets enriched by calcium, magnesium, and potassium, boosting biodiversity and crops.
In water, the rocks can be dissolved into seawater, making the ocean more capable of CO₂ absorption by increasing alkalinity and reducing acidification. This helps mitigate coral bleaching, and improves marine life of shellfish and corals.
Many pilot projects are being funded by environmental NGOs, climate funds, and governments, such as Coastal Carbon Capture by Vesta Earth. This project adds carbon-removing olivine sand to coastal systems, permanently storing carbon while reducing ocean acidity. They are working on developing carbon credits, with the market for OBCR in early stages, with scaled deployment expected within the next 10-20 years.
The method has limitations. It requires significant energy for the transportation and crushing of rocks, requiring renewable and sustainable operations. To have a significant global impact on acidification, the amounts of rock required would be immense, posing logistical and financial risks. Moreover, high contents of rock particles could disrupt and smother marine life, and some marine food chains would be impacted by changes in alkalinity.
Biochar Production
Biochar combines “biological” and “charcoal”. This living charcoal is an organic, sustainable source that offers many benefits to soil health and can sequester huge amounts of carbon.
It dates back over 2,500 years, with recorded uses by indigenous people in the Amazon Basin of South America for healthy soil purposes.
It can be made from materials like wood chips, straw, leaves, corn stover, as well as manure from poultry, pigs and cows. These are put through a process called pyrolysis where the oxygen is removed at a high temperature, triggering decomposition and resulting in biochar. The carbon is trapped inside in a stable form, effectively sequestering it for an extended period, and preventing it from adding to CO₂ emissions.
Biochar is incorporated into the soil before planting or during the soil’s preparation, ensuring soils will be fertile, healthy and able to retain high levels of nutrients to support maximum plant health.
Each ton of biochar is able to sequester around 2-3 tons of CO₂, and biochar carbon credits are being sold on platforms like Puro.earth and Carbon Direct at a rough average of $185 per ton of CO₂ removed.
Mineral Carbonation
In mineral carbonation, stable carbonate materials are formed when CO₂ reacts with minerals such as calcium or magnesium silicates that naturally occur. This chemically binds CO₂ into stable carbonate minerals, offering a permanent form of carbon sequestration and a durable, long-term solution.
CO₂ can be captured from industrial emissions or directly from the air, then reacted with crushed minerals either underground or in controlled facilities. Each ton of CO₂ mineralized can be measured in a straightforward way, leading to reliable and verifiable carbon credits.
The mineral carbonation can then be utilised for concrete or construction materials, where the carbon is locked in as part of the concrete’s structure.
Carbon Capture from Industrial Processes
This method involves carbon capture systems used at industrial sites like cement factories, chemical plants, steel mills, or power plants. The emissions from the sites are directly captured, and either reused or stored underground.
Technologies to capture emissions include pre-combustion (separating it from other gases), post-combustion (capturing CO₂ from flue gases after combustion), or oxy-fuel combustion (where the fuel is burned in pure oxygen).
The CO₂, once captured, can be compressed into a transparent liquid, where it can be injected into geological formations such as depleted oil and gas fields or saline aquifers. This is because deep underground, large volumes of CO₂ can be stored safely.
On the other hand, the liquid CO₂ can be utilised for other purposes, like carbonating drinks, or enhancing the performance of some metals.
Carbon Farming
Carbon farming is a range of agricultural practices specifically designed for the capture and storage of CO₂. They are strategically created to optimally store carbon in biomass, plants, and soil.
A method of this is livestock management, with rotational grazing for regenerated grasslands to allow for more carbon storage. With manure as soil, capacity for carbon storage increases.
Many regenerative agricultural practices are in line with carbon farming, such as agroforestry, where the combination of trees and crops allows for more carbon to be stored, or silvopastures, which encourage soil regeneration and biodiverse ecosystems.
More Information
https://www.gov.uk/guidance/renewable-and-low-carbon-energy
https://www.eea.europa.eu/en/topics/in-depth/climate-change-mitigation-reducing-emissions
https://www.sylvera.com/blog/renewables-carbon-credits-framework
https://www.irena.org/publications/2021/Aug/Bracing-for-climate-impact-2021
https://www.energy.gov/eere/bioenergy/renewable-carbon-resources